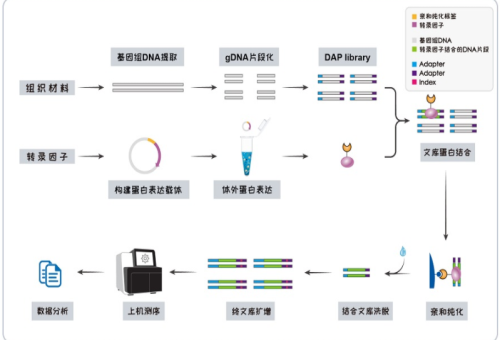
DAP-seq(DNA亲和纯化测序)
100+物种,2000+转录因子实战经验,无需抗体
高通量检测转录因子或DNA结合蛋白在基因组上的结合位点
已助力客户在许多期刊发表文章:Molecular Plant,The Plant Cell,Plant Physiology,Plant Biotechnology Journal,New Phytologist等
- 技术简介
- 服务列表
- 服务内容
- 经验分享
- 常见问题
- 客户文章
在功能基因组学和表观遗传学研究中,转录因子结合位点(TFBS)的发掘一直是研究热点。传统的ChIP-seq(染色质免疫共沉淀测序)方法,在抗体质量很好的情况下能够有效检测到TFBS。然而,好的抗体可遇不可求,这限制了ChIP-seq更广泛的应用。
DAP-seq技术的出现,使TFBS 的研究不再局限于物种,不再受抗体质量的限制,为生命科学领域转录因子的研究提供了新的有效工具。
DAP-seq与ChIP-seq技术对比
技术名称 | DAP-seq | ChIP-seq |
实验模式 | 体外 | 体内 |
是否需要特异性抗体 | 否 | 是 |
是否适用于非模式物种 | 是 | 否 |
时间成本 | 低 | 高 |
是否高通量 | 是 | 否 |
蓝景科信为您提供DAP-seq全流程技术服务和个性化数据分析,具有100多个物种,2000多个转录因子的实战经验,已助力客户在许多期刊发表文章,例如:Molecular Plant,The Plant Cell,Plant Physiology,Plant Biotechnology Journal,New Phytologist,Journal of Integrative Plant Biology等,欢迎咨询。
参考文献:
O'Malley RC, Huang SC, Song L, Lewsey MG, Bartlett A, Nery JR, Galli M, Gallavotti A, Ecker JR. Cistrome and Epicistrome Features Shape the Regulatory DNA Landscape. Cell. 2016. 165(5):1280-1292. doi: 10.1016/j.cell.2016.04.038.
2016-Cell-DAP Seq-Cistrome and Epicistrome Features Shape the Regulatory DNA Landscape.pdf
Bartlett A, O'Malley RC, Huang SC, Galli M, Nery JR, Gallavotti A, Ecker JR. Mapping genome-wide transcription-factor binding sites using DAP-seq. Nat Protoc. 2017. (8):1659-1672. doi: 10.1038/nprot.2017.055.
2017-Nature Protocols-Mapping genome-wide transcription-factor binding sites using DAP-seq.pdf
服务项目 | 周期 | 交付结果 | 报价 |
蛋白表达载体构建 | 1-2周 | 构建载体的测序结果 实验过程图 原始测序数据 分析结果 | 详细报价请电询400-6187099 或15632249798 |
蛋白无细胞表达 | 1-2周 | ||
DAP-seq文库构建 | 1周 | ||
DNA亲和纯化 | 1-2周 | ||
上机测序 | 2周 | ||
标准数据分析 | 2周 |
实验流程 |
生信分析 |
|
|
项目可行性分析 |
开展项目之前,我们会根据您具体的转录因子做可行性分析报告,供您参考,从多个方面进行可行性分析,包括转录因子分子量,亚细胞定位预测,跨膜区预测,蛋白质结构域预测、翻译后修饰预测,并且根据文献报道和我们的经验来进行可行性分析。
已做物种 | ||||||||
植物 | ||||||||
拟南芥 | 茎瘤芥 | 甘蓝型油菜 | 白菜型油菜 | 不结球白菜 | 菜心 | 小麦 | 大麦 | 花生 |
辣椒 | 番茄 | 草莓 | 黄花棘豆 | 苦荞 | 红薯 | 木薯 | 马铃薯 | 普通烟草 |
人参 | 鸭茅 | 罂粟 | 甘蔗 | 短芒大麦草 | 二色补血草 | 烟草 | 百脉根 | 芍药 |
丹参 | 狗尾草 | 菠菜 | 玉米 | 大豆 | 高粱 | 藜麦 | 陆地棉 | 甜瓜 |
黄瓜 | 葡萄 | 灰毡毛忍冬 | 粉葛 | 三叶青 | 猕猴桃 | 香蕉 | 蒺藜苜蓿 | 紫花苜蓿 |
伴矿景天 | 苔藓 | 地钱 | 毛果杨 | 717杨 | 84K杨 | 小黑杨 | 胡杨 | 山新杨 |
小叶杨 | 欧美杨 | 大青杨 | 毛白杨 | 刚毛柽柳 | 白桦 | 光皮桦 | 油松 | 毛竹 |
麻竹 | 银杏 | 油桐 | 荔枝 | 柑橘 | 甜橙 | 欧洲云杉 | 核桃 | 柿子 |
闽楠 | 木荷 | 脐橙 | 板栗 | 枣 | 枳 | 杜梨 | 苹果 | 桃 |
樱桃 | 麻疯树 | 茶树 | 梅 | 月季 | 海岛棉 | 白木香 | 橡胶树 | 三角褐指藻 |
芥蓝 | 蓝花耧斗菜 | 盐芥 | 无花果 | 菠萝 | 西瓜 | 甘薯 | 竹叶花椒 | 玫瑰 |
动物 | ||||||||
驴 | 飞蝗 | 新孢子虫 | 烟粉虱 | 草地贪夜蛾 | ||||
真菌 | ||||||||
拟轮枝镰孢菌 | 猪苓真菌 | 意大利青霉 | 草酸青霉 | 腐霉 | 金黄壳囊孢 | 灵芝 | 糙皮侧耳 | 草菇 |
灰盖鬼伞 | 虫草 | 亚洲镰刀菌 | 蝗绿僵菌 | |||||
细菌 | ||||||||
路德维希肠杆菌 | 嗜热厌氧杆菌 | 生氮假单胞菌 | 伯克赫尔德氏菌 | 布鲁氏菌 | 肺炎克雷伯菌 |
1.DAP-seq原理是什么,技术流程是什么,能帮我解决什么样的问题?
原理:体外表达的蛋白和DNA进行亲和纯化,将与蛋白结合的DNA洗脱后进行高通量测序。
技术流程:将编码转录因子的CDS序列构建到含有亲和标签的载体中,构建蛋白表达载体,进行体外蛋白表达,形成转录因子和亲和标签的融合蛋白;提取样品的基因组DNA,构建DNA文库,然后将体外表达的带有亲和标签的转录因子和DNA文库进行结合,随后把结合的DNA洗脱后上机测序。
能帮助您快速找到转录因子的结合位点,寻找转录因子调控的靶基因。
技术服务流程:
2.需要提供什么材料?
需要您提供
(1)组织材料或者是提取好的基因组DNA;
(2)含有转录因子CDS序列的质粒。
3.分析结果包括哪些内容?
蓝景科信DAP-seq的生信分析包括以下内容:
1. 对原始数据进行去除接头、污染序列及低质量 reads 的处理
2. 数据产出统计
3. 参考序列比对分析
4. 测序reads富集区域扫描(peak calling)
5. Peak在基因功能元件上的分布统计
6. Peak序列模式发掘(motif search)
7. 已知motif注释
8. Peak相关基因鉴定
9. Peak相关基因的GO和KEGG富集分析
10. 测序数据的可视化分析
4.实验的成功率怎么样?
不同转录因子家族的成功率不同,请参考不同转录因子家族的DAP-seq成功率:
不同转录因子家族成功率 | |||
转录因子 家族类型 | 该家族已做 转录因子的数量 | 成功鉴定到Motif的 转录因子数量 | 该家族转录因子的 成功率 |
C2H2 | 151 | 27 | 18% |
bHLH | 137 | 18 | 13% |
AP2-EREBP | 133 | 74 | 56% |
C3H | 129 | 9 | 7% |
MYB | 116 | 55 | 47% |
MADS | 86 | 10 | 12% |
NAC | 76 | 51 | 67% |
MYB-related | 71 | 26 | 37% |
WRKY | 65 | 34 | 52% |
ND | 60 | 5 | 8% |
Homeobox | 43 | 13 | 30% |
ABI3-VP1 | 40 | 7 | 18% |
bZIP | 38 | 29 | 76% |
G2-like | 37 | 17 | 46% |
LOB-AS2 | 35 | 8 | 23% |
Orphan | 35 | 3 | 9% |
C2C2-CO-like | 34 | 2 | 6% |
C2C2-DOF | 32 | 21 | 66% |
C2C2-GATA | 28 | 13 | 46% |
HB | 27 | 10 | 37% |
Trihelix | 27 | 13 | 48% |
TCP | 26 | 13 | 50% |
mTERF | 23 | 1 | 4% |
GeBP | 19 | 2 | 11% |
HSF | 17 | 10 | 59% |
SBP | 16 | 8 | 50% |
ZF-HD | 14 | 6 | 43% |
ARF | 12 | 3 | 25% |
CCAAT-HAP5 | 12 | 2 | 17% |
FAR1 | 12 | 1 | 8% |
FHA | 12 | 1 | 8% |
HMG | 12 | 1 | 8% |
CCAAT-HAP3 | 11 | 2 | 18% |
PLATZ | 11 | 1 | 9% |
ARID | 10 | 5 | 50% |
LIM | 10 | 1 | 10% |
BSD | 9 | 1 | 11% |
CPP | 8 | 4 | 50% |
GRF | 8 | 2 | 25% |
REM(B3) | 8 | 1 | 13% |
SRS | 8 | 1 | 13% |
BBR/BPC | 7 | 3 | 43% |
E2F-DP | 7 | 4 | 57% |
BZR | 6 | 4 | 67% |
C2C2-YABBY | 6 | 1 | 17% |
CAMTA | 5 | 2 | 40% |
EIL | 5 | 2 | 40% |
REM | 5 | 1 | 20% |
DBP | 4 | 1 | 25% |
NLP | 4 | 1 | 25% |
RAV | 4 | 1 | 25% |
RWP-RK | 4 | 2 | 50% |
S1Fa-like | 3 | 1 | 33% |
BES1 | 2 | 1 | 50% |
zf-GRF | 1 | 1 | 100% |
此表数据来源文献,doi: 10.1016/j.cell.2016.04.038。
5.为什么有些基因家族的成功率很低?
有些转录因子需要和其他蛋白形成复合体才能与DNA结合,这些蛋白的风险比较高。
6.一些特殊的样品能不能做,有没有风险?
有两种情况的样品是不能做DAP-seq 实验的,一种情况是没有参考基因组,另一种情况是转录因子不能在体外表达出来,除此之外,我们会做可行性分析报告供您参考。
7.包含重复吗?
包含两个技术重复。
8.做这个蛋白表达的时候,使用的什么表达系统?
优先使用真核表达系统进行蛋白表达,如果真核表达系统不能表达成功的话可以沟通换用原核表达系统。
9.植物组织样本取样的时期部位有什么要求?
植物组织样本取样的时期和部位是您根据自己的研究需求确定,不同组织和时期DNA的修饰不同,可能会影响蛋白和DNA的结合。
10.DAP-seq试验结果的可靠性如何,是否能通过验证试验做出来?
可以参考2019-JXB-Populus euphratica PeWRKY1 binds the promoter of H+-ATPase gene to enhance gene expression and salt tolerance这篇文献,文献中是使用DAP-seq技术,在基因组水平上,鉴定了PeWRKY1转录因子与胡杨基因组DNA的结合位点信息,并通过酵母单杂交、EMSA、荧光素酶检测系统验证了这一结果。
11.DAP-seq跟ChIP-seq有何区别,DAP-seq的优势表现在哪里?
DAP-seq和ChIP-seq的区别:
DAP-seq的优势:不需要针对每个转录因子制备特异性抗体,快速、高通量、节约时间成本。
12、DAP-seq用的input是什么,为什么选这个作为对照呢?
Input对照是用的亲和纯化前的文库,目的是降低背景噪音,我们用的Input和2016年发表在Cell(DAP Seq-Cistrome and Epicistrome Features Shape the Regulatory DNA Landscape)上的论文是一致的。
13、为什么实验中表达的有些蛋白比理论值偏大?
很多蛋白表达出来比理论值大一些,因为有一些翻译后修饰,很多情况都是这样的,原核表达也有这类情况,比如拟南芥SnRK蛋白激酶,预测40 kd,通过原核表达,实际分子量是60 kd。
题目 | 期刊 | IF | 发表日期 |
Arabidopsis WRKY1 promotes monocarpic senescence by integrative regulation of flowering, leaf senescence and nitrogen remobilization | Mol Plant | 17.1 | 2024.7.13 |
PaMYB11 promotes suberin deposition in Norway spruce embryogenic tissue during cryopreservation: A novel resistance mechanism against osmosis | Plant J | 6.2 | 2024.7.11 |
Transcription factor OsbZlP10 modulates rice grain quality by regulating OsGIF1 | Plant J | 6.2 | 2024.7.9 |
The MdHSC70-MdWRKY75 module mediates basal apple thermotolerance by regulating the expression of heat shock factor genes | Plant Cell | 10 | 2024.6.12 |
MdVQ17 negatively regulates apple resistance to Glomerella leaf spot by promoting MdWRKY17-mediated SA degradation and pectin lyase activity | Hortic Res | 7.6 | 2024.6.7 |
Abscisic acid controls sugar accumulation essential to strawberry fruit ripening via the FaRIPK1-FaTCP7-FaSTP13/FaSPT module | Plant J | 6.2 | 2024.5.30 |
The MdVQ37-MdWRKY100 complex regulates salicylic acid content and MdRPM1 expression to modulate resistance to Glomerella leaf spot in apples | Plant Biotechnol J | 10.1 | 2024.4.29 |
Contribution of the transcription factor SfGATAe to Bt Cry toxin resistance in Spodoptera frugiperda through reduction of ABCC2 expression | Int J Biol Macromol | 7.7 | 2024.4.7 |
GhRCD1 promotes cotton tolerance to cadmium by regulating the GhbHLH12-GhMYB44-GhHMA1 transcriptional cascade | Plant Biotechnol J | 10.1 | 2024.2.13 |
AvERF73 positively regulates waterlogging tolerance in kiwifruit by participating in hypoxia response and mevalonate pathway | Hortic Plant J | 5.7 | 2024.2.2 |
ETHYLENE RESPONSE FACTOR 070 inhibits flowering in Pak-choi by indirectly impairing BcLEAFY expression | Plant Physiol | 6.5 | 2024.1.25 |
The transcription factor MdBPC2 alters apple growth and promotes dwarfing by regulating auxin biosynthesis | Plant Cell | 11.6 | 2023.11.29 |
Transcription factor PpNAC1 and DNA demethylase PpDML1 synergistically regulate peach fruit ripening | Plant Physiol | 7.4 | 2023.11.22 |
GhRCD1 regulates cotton somatic embryogenesis by modulating the GhMYC3-GhMYB44-GhLBD18 transcriptional cascade | New Phytol | 9.4 | 2023.7.11 |
ZmEREB57 regulates OPDA synthesis and enhances salt stress tolerance through two distinct signalling pathways in Zea mays | Plant Cell Environ | 7.3 | 2023.6.16 |
Identification of the target genes of AhTWRKY24 and AhTWRKY106 transcription factors reveals their regulatory network in Arachis hypogaea cv. Tifrunner using DAP-seq | Oil Crop Science | 2023.6.4 | |
Allelic variation in transcription factor PtoWRKY68 contributes to drought tolerance in Populus | Plant Physiol | 7.4 | 2023.5.29 |
Overexpression of the transcription factor MdWRKY115 improves drought and osmotic stress tolerance by directly binding to the MdRD22 promoter in apple | Hortic Plant J | 5.7 | 2023.5.22 |
Control of ovule development in Vitis vinifera by VvMADS28 and interacting genes | Hortic Res | 8.7 | 2023.4.13 |
PuCRZ1, an C2H2 transcription factor from Polyporus umbellatus, positively regulates mycelium response to osmotic stress | Front Microbiol | 5.2 | 2023.4.6 |
A transcription factor of the NAC family regulates nitrate-induced legume nodule senescence | New Phytol | 9.4 | 2023.3.22 |
Single-cell transcriptomic analysis reveals the developmental trajectory and transcriptional regulatory networks of pigment glands in Gossypium bickii | Mol Plant | 27.5 | 2023.2.9 |
MdERF114 enhances the resistance of apple roots to Fusarium solani by regulating the transcription of MdPRX63 | Plant Physiol | 7.4 | 2023.1.31 |
The bHLH-zip transcription factor SREBP regulates triterpenoid and lipid metabolisms in the medicinal fungus Ganoderma lingzhi | Commun Biol | 5.9 | 2023.1.3 |
A brassinosteroid transcriptional regulatory network participates in regulating fiber elongation in cotton | Plant Physiol | 8.005 | 2022.12.21 |
Enhanced genome-wide association reveals the role of YABBY11-NGATHA-LIKE1 in leaf serration development of Populus | Plant Physiol | 8.005 | 2022.12.19 |
The NAC transcription factor ONAC083 negatively regulates rice immunity against Magnaporthe oryzae by directly activating transcription of the RING-H2 gene OsRFPH2-6 | J Integr Plant Bio | 9.106 | 2022.10.29 |
CC-type glutaredoxin, MeGRXC3, associates with catalases and negatively regulates drought tolerance in cassava (Manihot esculenta Crantz) | Plant Biotechnol J | 13.263 | 2022.9.1 |
ScAIL1 modulates plant defense responses by targeting DELLA and regulating GA and JA signaling | J Exp Bot | 7.298 | 2022.8.20 |
OsSGT1 promotes melatonin-ameliorated seed tolerance to chromium stress by affecting the OsABI5-OsAPX1 transcriptional module in rice | Plant J | 7.091 | 2022.8.9 |
Phytochrome Interacting Factor Regulates Stomatal Aperture by Coordinating Red Light and Abscisic Acid | Plant Cell | 12.085 | 2022.8.5 |
A newly characterized allele of ZmR1 increases anthocyanin content in whole maize plant and the regulation mechanism of diferent ZmR1 alleles | Theor Appl Genet | 5.574 | 2022.7.5 |
Rice CIRCADIAN CLOCK ASSOCIATED1 transcriptionally regulates ABA signaling to confer multiple abiotic stress tolerance | Plant Physiol | 8.005 | 2022.5.4 |
Genome-Wide Identification of Direct Targets of ZjVND7 Reveals the Putative Roles of Whole-Genome Duplication in Sour Jujube in Regulating Xylem Vessel Differentiation and Drought Tolerance | Front Plant Sci | 6.627 | 2022.2.4 |
A R2R3-MYB transcriptional activator LmMYB15 regulates chlorogenic acid biosynthesis and phenylpropanoid metabolism in Lonicera macranthoides | Plant Sci | 4.729 | 2021.4.29 |
Growth-regulating factor 5 (GRF5)-mediated gene regulatory network promotes leaf growth and expansion in poplar | New Phytol | 10.151 | 2021.1.10 |
Phytochrome-interacting factors regulate seedling growth through ABA signaling | BBRC | 2.985 | 2020.4.16 |
Populus euphratica WRKY1 binds the promoter of H+-ATPase gene to enhance gene expression and salt tolerance | J Exp Bot | 5.36 | 2019.11.4 |